Scientists unravel the secret ‘switch’ that makes antibiotic-resistant bacteria go incognito
Microbiologists are uncovering the mechanism that makes antibiotic-resistant bacteria undetectable by standard lab tests. Now, Kristel Tjandra reports on this incredible evolutionary feat and how it may change our perception of infections. Illustrated by Amanda Konishi.
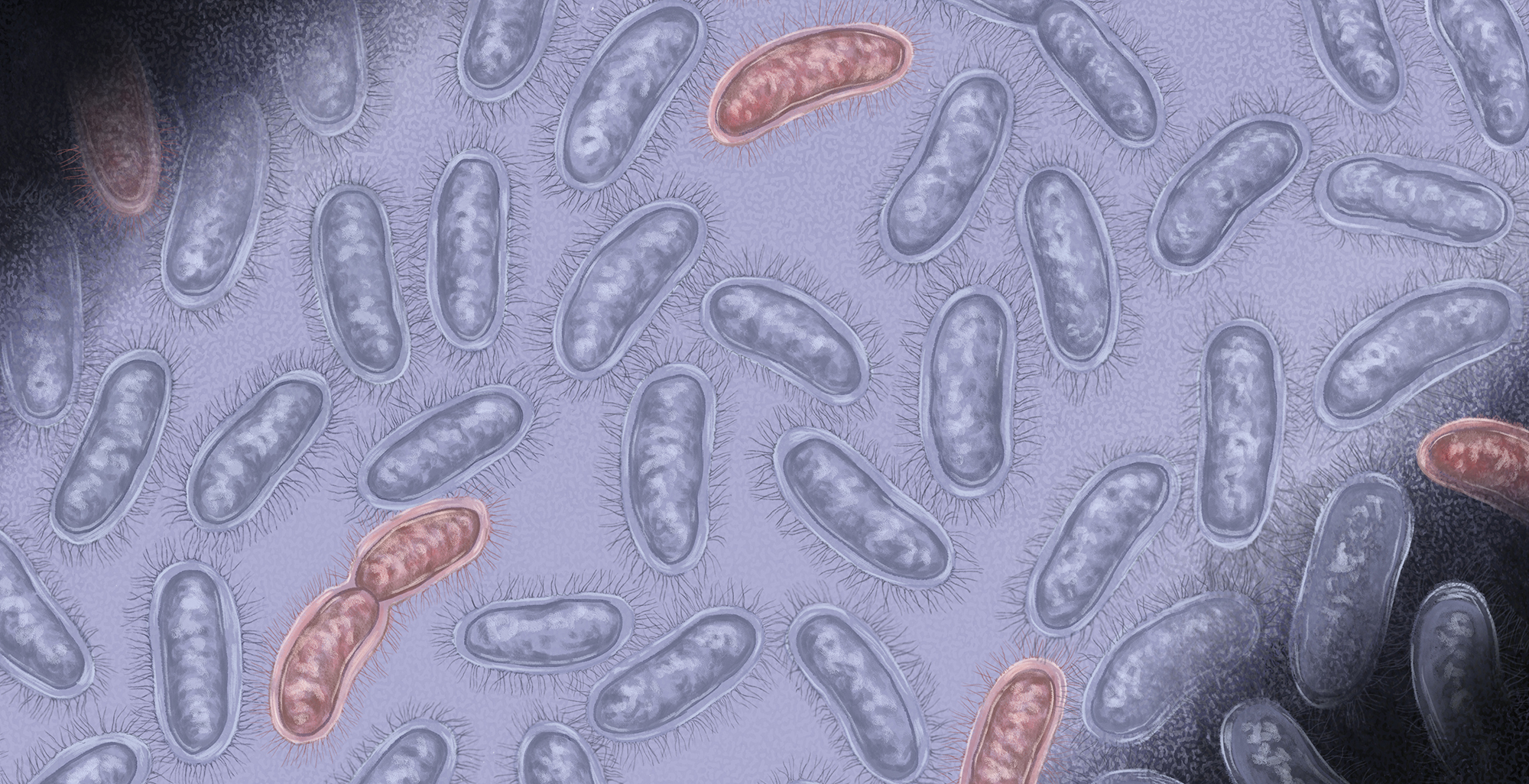
Illustration: Amanda Konishi
I n 2021, a patient arrived at Emory University Hospital in Atlanta, Georgia with a life-threatening infection from an unusual bug. The bacteria, Klebsiella pneumoniae, were resistant to all first-line antibiotics, making it difficult to treat. After running several tests, the Emory doctors finally found a drug that worked against the bacteria. They prescribed the man an antibiotic called cefiderocol. He took it for two weeks and his infection appeared to clear. Blood analysis even confirmed he was bacteria-free.
But just ten days later, the man fell ill again. His blood pressure dropped, and he was rushed into the intensive care unit, where the doctors discovered a pus-filled mass covering his liver. To their surprise, the same bacteria had returned with a vengeance.
The doctors sent the patient’s blood sample to David Weiss, a microbiologist at Emory Antibiotic Resistance Center. After a more thorough analysis, Weiss discovered that the bacteria were highly resistant to the antibiotic cefiderocol. But their puzzling survival strategy is almost impossible to detect in standard lab tests.
Weiss quickly notified the doctors. But even after they treated the man with a different antibiotic, he didn’t survive.
Cases like this are not always documented or published in a medical journal but evidence of treatment failure from unusual antibiotic resistance is emerging, said Weiss. Bacteria have developed remarkable ways to evade antibiotics. With the lack of new antibiotics, infections that used to be easy to treat could become life-threatening. Each year, antibiotic resistance claims approximately 5 million lives. It is a medical nightmare. When a person gets a bacterial infection, doctors send their blood samples to microbiologists to test which antibiotic would kill the pathogen. The bacteria that survive a certain dose of antibiotic are considered resistant, while those that succumb to it are susceptible. However, as Weiss and other scientists now realized, not all bacteria fit into this binary ‘susceptible or resistant’ category. And that could complicate the way doctors detect and treat infections.
For decades, microbiologists like Weiss thought of antibiotic resistance as something a single species of bacteria either had or didn’t have. But he says, “now, we are realizing that that’s not always the case.” Klebsiella pneumoniae, the bacteria that killed the patient at Emory, is what microbiologists call ‘heteroresistant’. These bacteria are genetic shapeshifters.
Normally, there is a clear genetic basis for how bacteria resist certain antibiotics. For example, bacteria could gain a gene mutation that enables them to modify the antibiotics and render these drugs ineffective. In other cases, a genetic mutation could equip the bacteria with the necessary proteins that prevent the drugs from breaking into their cell walls. In most cases, resistance genes are permanently inscribed into the DNA of the bacteria like a protective armory that they always wear. And when circumstances arise, these armories are ready to protect them.
But that is not the case for heteroresistant bacteria.
These bacteria could defeat the drugs designed to kill them without leaving a mark on their genome that indicates their resistances. In other words, they lack bona fide resistant genes. When the antibiotic is not present, these bacteria could stow away their resistance armories and dress like any other bacteria.
When typical antibiotic-resistant bacteria propagate, their resistance genes are passed down to the next generation, creating a legion of antibiotic-resistant populations that collectively counter the treatment. Meanwhile, the bacteria in a heteroresistant population are divided in their antibiotic responses. Most of these bacteria are sensitive to antibiotics. But, at certain doses of antibiotic, a small proportion of this heteroresistant population – as little as one in a million – could unpredictably turn antibiotic-resistant and survive the drugs, while the rest of the population stop growing and die.
As if hiding themselves in an invisible cloak, the population of resistant cells in a heteroresistant population blends in with these antibiotic-susceptible cells, making it hard for microbiologists to detect these resistant cells.
Failing to detect antibiotic resistance is a high-stakes error that can lead to treatment failure and even fatal outcomes. Scientists across the globe have spent years looking for answers as to why and how heteroresistance develops so that they can better detect them. Now, new clues are finally beginning to emerge.
Face-to-face with the rogue bacteria
For Karin Hjort, her first encounter with heteroresistance happened serendipitously, when a test tube full of frozen bacteria in her lab went rogue. About ten years ago, Hjort, a microbiologist at Uppsala University, Sweden, was growing a host of bacteria. Strain by strain, she administered lethal doses of antibiotics, labeling any survivors as ‘resistant’ and freezing them. When she thawed out the survivors, she searched for abnormal genetic changes, known as mutations, that typically underlie their ability to cheat a certain death.
But this time was different.
“I took them out of the freezer, I sequenced them and sequenced them, and I didn’t find any mutations,” said Hjort.
“The resistance was lost in the freezer,” she said.
“Everyone called out ‘heteroresistant’ in different ways –there was no standard to look at.”
Hjort couldn’t figure out what was happening then, but as she flipped through the literature, she found hints in a 1970 research paper. In it, scientists at the University of California, Davis reported a phenomenon they called ‘heteroresistance’ in which a population of bacteria that were mildly susceptible to an antibiotic became completely resistant to the same antibiotic at an unusually fast rate. The phenomenon caught the attention of the research community, but reports about heteroresistance remained sparse and vague, Hjort said.
While ‘heteroresistance’ was first mentioned back in the 1940s, the phenomenon has remained very challenging to study and its origin is shrouded in mystery. Without a clear definition, scientists struggle to compare their observations.
“Everyone called out ‘heteroresistant’ in different ways –there was no standard to look at,” said Omar El-Halfawy, a microbiologist and Canada Research Chair in Chemogenomics and Antimicrobial Research at the University of Regina, Canada. Some people use the term ‘heteroresistance’ to describe a situation whereby multiple types of bacteria that have different tolerance levels to an antibiotic co-infected a person. Others used it to describe a situation when two different antibiotic susceptibility tests gave different results.
Determined to settle the issue, in 2015 El-Halfawy reviewed every study he could find that described instances of heteroresistance in pursuit of a more suitable definition. Ultimately, he proposed defining heteroresistance as a population of bacteria from a bacteria culture where a fraction of the population can resist a much higher antibiotic concentration than the rest of the population. Scientists adopted this definition and the number of peer-reviewed publications on heteroresistance has doubled.
Fooling the test
The number of resistant bacteria in a heteroresistant population can be anywhere between 1 in 10,000 to 1 in a million of the cells in the entire population. It is so rare that standard clinical microbiology tests would easily miss it.
“Essentially what you’re trying to do is detect a resistant needle in a susceptible haystack of cells,” said Weiss.
To put it into perspective, a football stadium can host between 60,000 to 80,000 spectators. Imagine having to spot 1 person when the stadium is full. That’s about as hard as it is to spot resistant cells in a heteroresistant population.
While it is not uncommon for bacteria to transition from being antibiotic-susceptible to antibiotic-resistant, this transition usually happens in small increments and over at least several days. With heteroresistance, the transition is almost immediate.
The switch between susceptible to resistant, and back to susceptible confuses test analyses.
“Every time you grow a strain, it’s going to grow a little bit differently. On one day it might come up as resistance, on the next day it might be susceptible,” Weiss said. So much so that, “having inconsistent, discrepant testing results was like a hallmark of the strain being heteroresistant,” he said.
The standard antibiotic susceptibility test relies on one principle: A population of bacteria, at a fixed density of about 10,000 to 100,000 cells in a milliliter of broth, is dosed with different concentrations of an antibiotic until they stop multiplying or die.
But, according to Hjort, this process is fundamentally inadequate to spot heteroresistance. Because these standard tests are designed to detect a population-wide behavior, something like heteroresistance, which is extremely rare, is easily missed. “The error is huge, even if we use more bacteria,” she said.
A few years ago, a scientist in the lab of Trinad Chakraborty at Justus-Liebig-Universität Gieße, encountered the heteroresistance conundrum in his lab result. She repeated a routine antibiotic susceptibility test six times and got a different result each time.
That’s not supposed to happen. Normally, when testing for antibiotic susceptibility, microbiologists can identify a clear threshold at which the antibiotic causes the bacteria to stop growing or die. But this time, the pattern is not clear-cut.
“We find that isolates will grow at two, four, and eight micrograms (of antibiotics). And then at 16, they’re gone; 32, they’re gone; and at 64 something is growing,” said Chakraborty, who is now a retired professor of microbiology.
Because it is unclear which concentration worked, it’s hard to make the call.
“It’s like a lottery,” he said.
Chakraborty later found that, rather than having a gene that makes them permanently resistant to the antibiotic, these bacteria activate their resistance mechanism as they sense changes in their environment.
So far, the only reliable method to spot heteroresistance is called population analysis profiling, or PAP. To perform this test, microbiologists grow the bacteria overnight and then place some of them on a series of Petri dishes containing different concentrations of antibiotics and monitor their growth. Because PAP covers a range of bacterial densities, the test can pick up patterns not normally detected by standard tests.
But the method is very laborious. On a given day, a microbiologist needs to prepare multiple petri dishes to test just one antibiotic against one strain of bacteria. When the number of variables, such as the type of antibiotic or the cell densities are added to the equation, the number of dishes triples or quadruples.
“If you do a big experiment, it can be 200 plates to count,” said Sofia Jonsson, a graduate student at Uppsala University who works with Hjort. For each plate or petri dish, she’d count hundreds of little dots that represent a colony of bacteria. “You become a really fast counter,” she said.
Because it is manually-intensive, PAP is not routinely done in the clinical setting to test for antibiotic susceptibility, even though it is the only reliable way to confirm a heteroresistant phenomenon.
“We need something that can detect this faster, more reliable, less costly, and less time-consuming,” Hjort said.
Weiss speculated that to detect heteroresistance, future tests would need to monitor bacteria at a single-cell level and have a one-in-a-million cell resolution. Some research is already making strides in that direction, he said. But none of these developments have reached the clinic yet.
Cracking the case
Since heteroresistance occurs only phenotypically, that is, without permanent genetic changes, “we want to know if there could be a genotype behind it,” Hjort said.
From Hjort’s observation, two groups of bacteria could have at least an eight-fold difference in antibiotic tolerance within a heteroresistant population. For example, if it takes two micrograms of antibiotics to kill most of the susceptible population, it will take at least 16 micrograms to kill the resistant ones.
To crack the case of the underpinning mechanism, she tested a handful of multi-drug resistant bacteria against 28 different antibiotics. Among the bacteria she tested were gram-negative Escherichia coli, Salmonella enterica, Klebsiella pneumoniae, and Acinetobacter baumannii species. This group of bacteria has an outer membrane that shields them from toxic substances. Not a single new class of antibiotic that targets gram-negative bacteria has been approved in the last 50 years, which adds to the urgency for microbiologists to understand how their resistance mechanisms work.
Using a whole-genome sequencing technique, Hjort found that these bacteria’s heteroresistance tendencies arose from spontaneous mutation tandem amplification, where a selection of genes is temporarily increased to help bacteria cope with a certain threat. In essence, the bacteria do not need a new mutation to resist the antibiotic, but simply produce more proteins from existing genes that have protective features.
Gene amplification, Hjort says, “is something you don’t usually look at.” Therefore, it is rarely detected, she said.
Earlier this year, Hjort expanded the study to include Gram-positive bacteria, Staphylococcus aureus, that causes common skin infections. These bacteria have no outer membranes and have different resistance mechanisms to that of Gram-negative strains.
Hjort had received 40 patient samples containing S.aureus that were isolated from hospital patients in Denmark, Norway, Spain, and Sweden. She grew the bacteria in the lab and tested their susceptibility against six antibiotics. When tested with a standard antibiotic susceptibility test, all these bacteria fell under the ‘susceptible’ category, meaning that any of these antibiotics would’ve qualified as being a treatment option for the infection. However, the PAP result suggested otherwise.
To her surprise, the bacteria showed heteroresistance to more than half of these antibiotics. When Hjort probed the genetics of these bacteria she found that the genes typically associated with antibiotic resistance were absent. Unlike the gram-negative bacteria, Hjort also found no signs of spontaneous tandem gene amplifications in these bacteria. Instead, she saw that the heteroresistance tendency correlated with changes in single base pairs on various genes, known as chromosomal point mutation. But the reason why these isolates show a heteroresistance tendency to only certain drugs is still a mystery.
Because the mechanism behind heteroresistance can be different across species and antibiotics, Hjort thinks that their treatment strategies ought to be different.
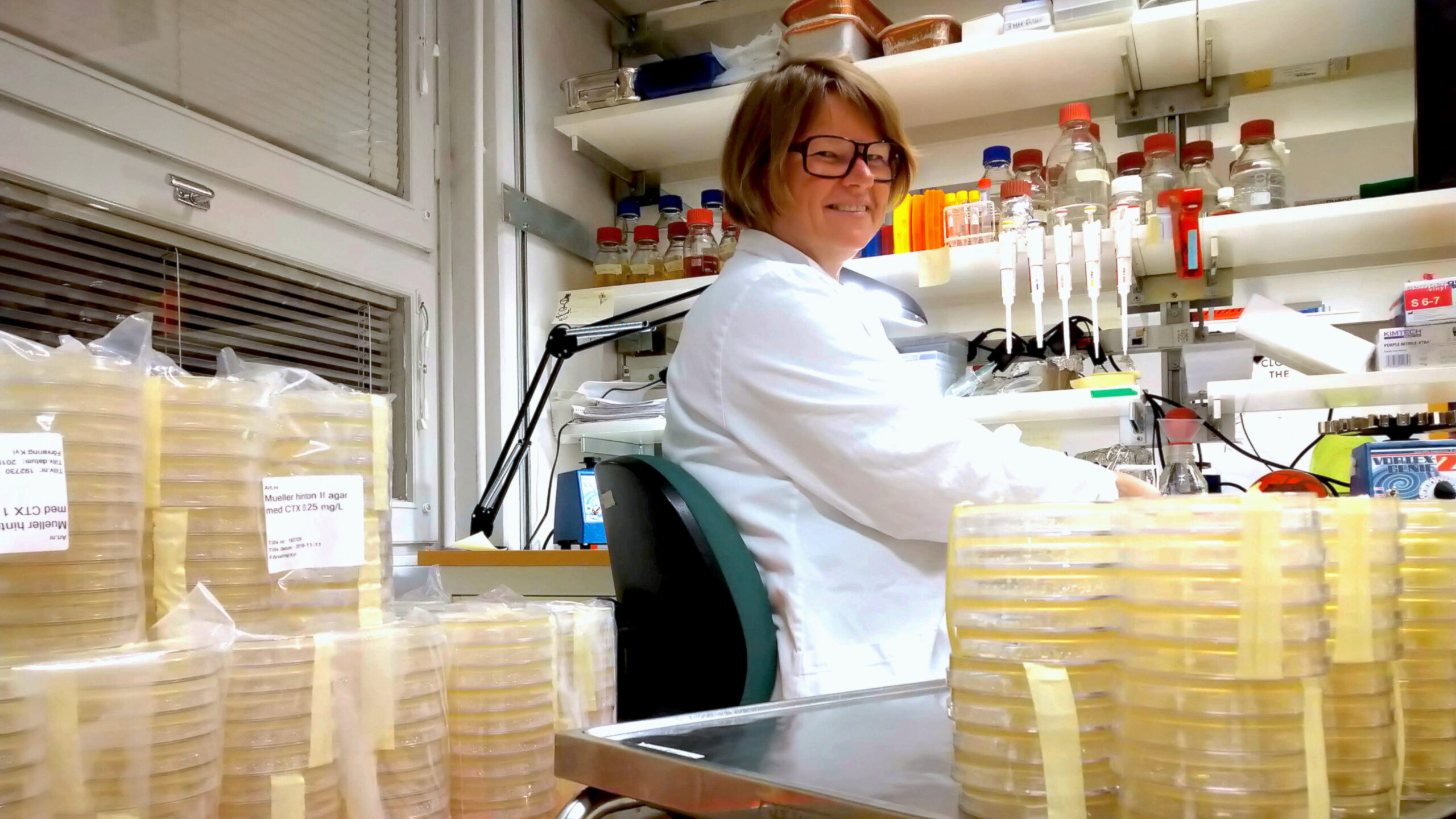
Karin Hjort performed routine population analysis profiling to identify heteroresistant bacteria in the lab. (Courtesy: Karin Hjort)
The quest continues
Scientists have now reported heteroresistance in almost every class of antibiotics. Matteo Bassetti, infectious disease physician and chair of the European Society of Clinical Microbiology and Infectious Diseases, said heteroresistant infection could create a dire situation, especially in cases where antibiotic options are lacking.
“The patient gets sicker and sicker. And you can only try to push on the dosage of the antibiotics or try to combine antibiotics with different mechanisms of action,” Bassetti said. “It is really, really, really difficult for the patients.”
While new clues have emerged, Hjort thinks that a much larger clinical dataset is needed to fully understand the link between heteroresistance and patient outcomes.
“It’s always better to know your enemy.”
“I want to dig deeper into heteroresistance. I want to find the mechanism and how it varies between different bacteria, how it affects treatments for patients, and if we can find a better way to detect it,” said Hjort.
Hjort believes that a good way to start is with a proper understanding of how these bacteria develop their heteroresistance in the first place.
Weiss agreed. By knowing the mechanism of action, “we may be able to develop new drugs that can reverse the heteroresistance and make the bacteria susceptible to the original drug,” he said.
Despite the potentially alarming reality, Weiss continues to be fascinated by bacterial complex biology. “It’s like uncovering a world that we didn’t know was there,” he said.
He is hopeful that the progress he and other scientists are making will help scientists find better approaches to managing the potential threat of heteroresistant bacteria.
“It’s always better to know your enemy,” he said.
A version of this article was published on the Live Science website in August 2024, and can be found here.
© 2024 Kristel Tjandra / UC Santa Cruz Science Communication Program
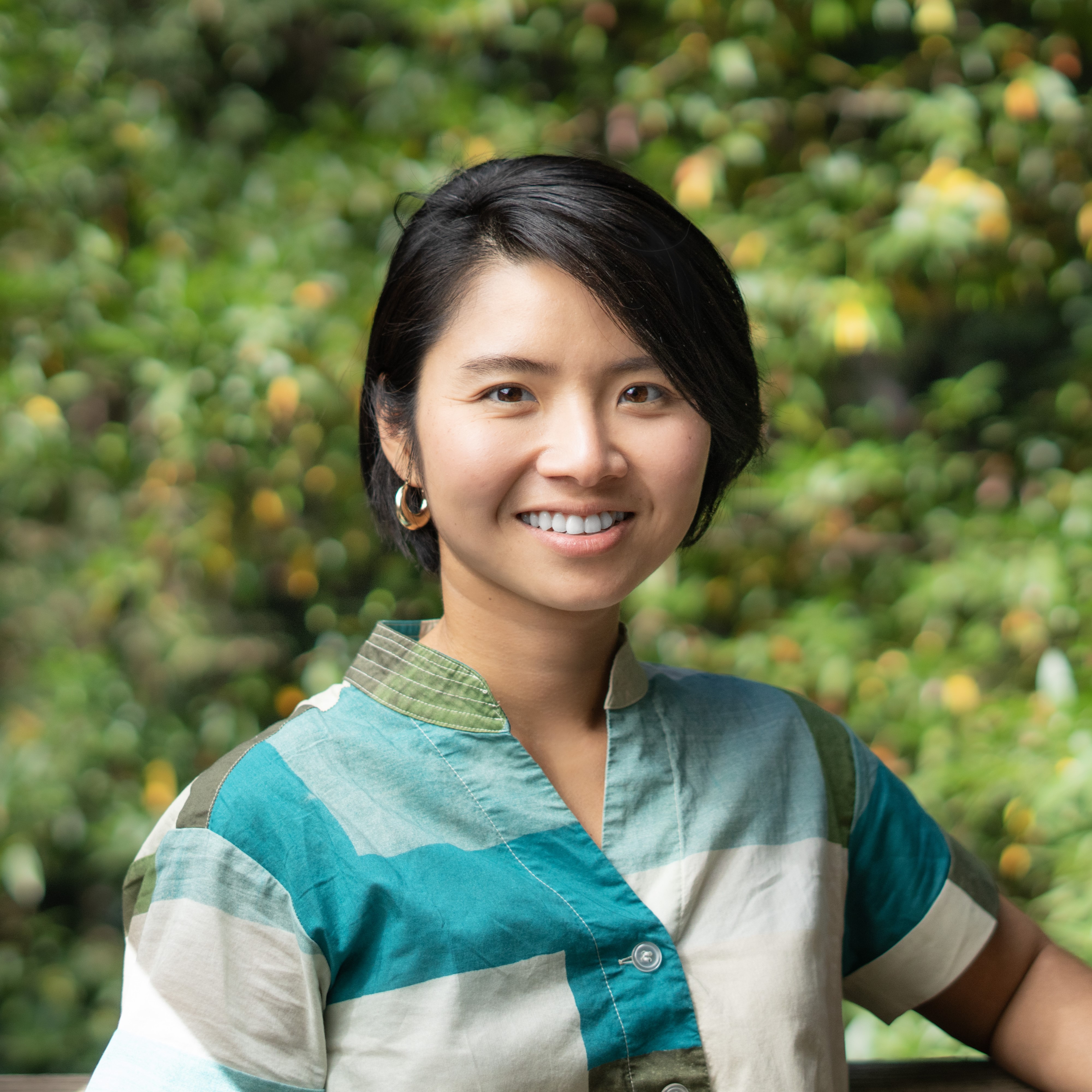
Kristel Tjandra
Author
Ph.D. (Chemistry) University of New South Wales, Australia
B.Med.Chem. University of New South Wales, Australia
Internships: Monterey Herald, KQED, Live Science
I met my first scientist in a children’s book. She was a Polish woman with two Nobel Prizes. Yes, you guessed it: Her name was Marie Curie. I saw the wonder of science through stories like hers, but the thought of being a scientist myself seemed like a fairy tale. After all, I didn’t know any real-life scientists. But when an opportunity arose for me to study chemistry, I took it without hesitation. For the next 10 years, I ventured from one laboratory to another, writing my own science story. Along the way, I encountered many other scientists whose unique and often inspiring stories were never told. By telling these stories, I hope to help others experience the everyday wonder of science, instead of thinking of science breakthroughs as a distant fairy tales.
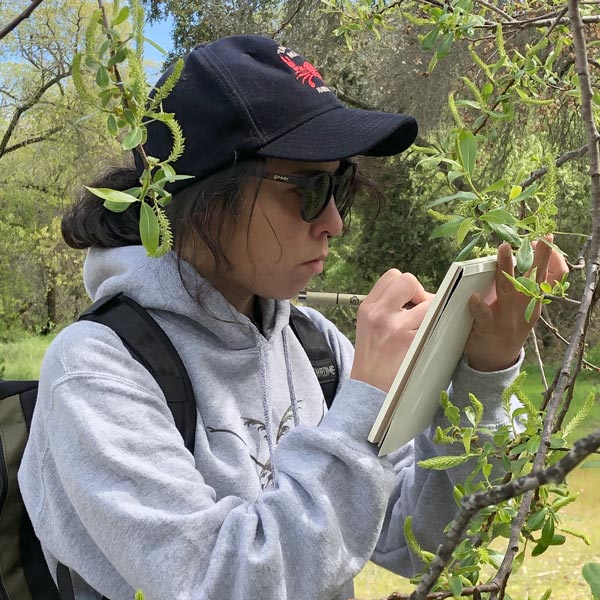
Amanda Konishi
Illustrator
B.F.A (Illustration) School of Visual Arts
Internship: Ink Dwell Studio (Berkeley, California)
Amanda is a painter and science illustrator based in New York and Marina, California. Born and raised in Southern California, she always placed great importance on the natural world as a source of inspiration. Having maintained a dedicated fine art studio practice for many years, her work in science illustration has led to a fascination with native plant and insect relationships, especially as seen in gall-forming insects and their hosts. Konishi’s work focuses on honoring the small environments around us, with an interest in community engagement through the intersection of art and science.