From warm little ponds to life on Mars
Understanding where life began on Earth could guide the search for life beyond our planet, Jack J. Lee reports. Illustrations by Hannah Caisse, Becca Hoskins and Madelyn Neufeld.
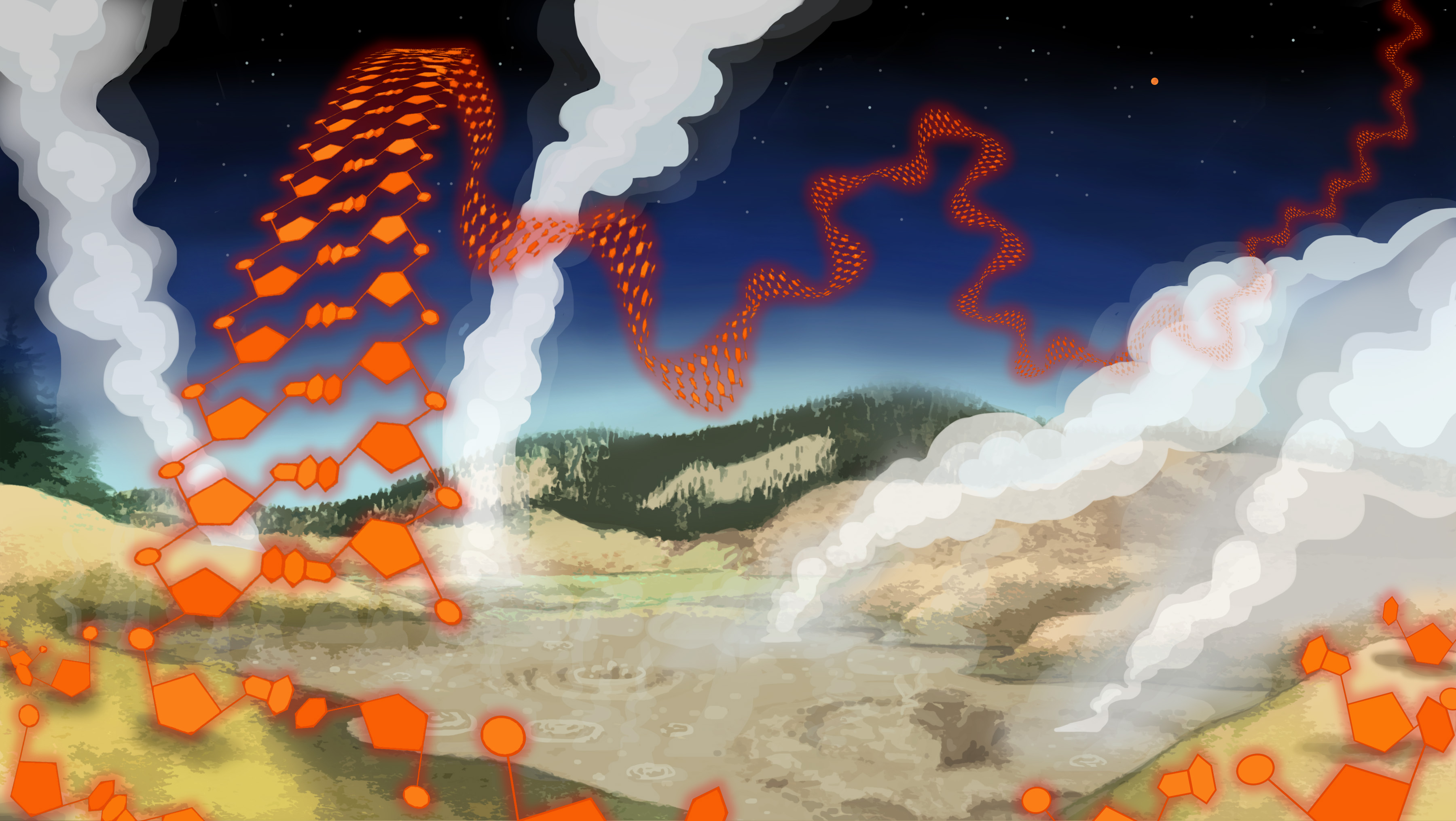
Illustration: Hannah Caisse
Bumpass Hell in Lassen Volcanic National Park seems like an unlikely place for life to survive, let alone thrive. A distinct aroma of rotten eggs fills the air. The ground is literally boiling: heated gas bubbles up through puddles of mud, producing goopy popping sounds. Fumaroles fire jets of scorching-hot steam out of the earth; vapor from one vent was once measured at 322 degrees Fahrenheit. The site was named for the cowboy Kendall Bumpass, who, in 1865, stepped through the thin crust and burned his leg so badly in boiling acidic water that it had to be amputated.
Yet some scientists think life on our planet arose in these apparently inhospitable conditions. Researchers at UC Santa Cruz and NASA’s Ames Research Center are exploring whether hot springs may have promoted chemical reactions that linked together simple molecules; the resulting chains may have been a first step toward complexity. This hypothesis contrasts with a widespread view that life first arose at hydrothermal vents, where heated mineral-rich water billows from cracks in the ocean floor.
In the field and in the lab, scientists are trying to recreate the conditions in which life may have originated. Their findings may help them address whether life started in hot springs or in the depths of the ocean. Answering that question could give hints about where life might be found beyond Earth, says Natalie Batalha, professor of astronomy and astrophysics at UC Santa Cruz and director of the Astrobiology Initiative: “It has very significant implications for the future of space exploration.”
Life in warm little ponds
In 1871, Darwin shared his thoughts about how life began in a letter with his close friend, J.D. Hooker: “But if (& oh what a big if) we could conceive in some warm little pond with all sorts of ammonia & phosphoric salts, —light, heat, electricity &c present, that a protein compound was chemically formed, ready to undergo still more complex changes…,” he wrote.
Darwin’s letter presciently predicted research published in 1953 by Stanley Miller. Based on discussions with Harold Urey, Miller simulated the effect of lightning on a combination of gases — methane, ammonia, hydrogen and water vapor — meant to reflect the atmosphere of early Earth. Sending electric sparks into the mixture produced a red, cloudy soup containing a handful of amino acids, the building blocks of proteins. Scientists now think early Earth’s atmosphere was primarily composed of nitrogen and carbon dioxide, rather than the mix Miller used, but electrical discharge experiments on other gaseous mixtures have similarly produced amino acids.
Fast-forwarding through several billion years of evolution, these amino acids have given rise to complex life, from single-celled microbes to humans. And the warm little ponds that Darwin described continue to be home to hardy examples of life: modern-day microbiologists have uncovered organisms that thrive in hot springs. In a 2016 study, for example, researchers described microbes living in water collected near the clay banks of Great Boiling Spring in Nevada.
“They are most likely closely related to some of the organisms that were originally on this planet,” says Jennifer Pett-Ridge, a research scientist at Lawrence Livermore National Laboratory. In addition to bacteria, some of the microbes are archaea, one of the three branches of life that are thought to represent some of the most ancestral life forms on Earth.
Archaea and bacteria can form communities known as microbial mats. These layers appear in a variety of environments, including geothermal areas like Yellowstone National Park and Lassen Volcanic National Park — where Bumpass Hell is located. Over time, microbial mats can produce stromatolites, stratified fossils where layers of microbes and minerals accumulate on top of one another; the layered appearance of a stromatolite is akin to growth rings in a tree.
In a 2017 study, Tara Djokic and colleagues investigated the Dresser Formation in Pilbara, Australia, a region that contained hot springs 3.5 billion years ago. They found evidence of stromatolites and suggest that microbes may have been present, indicating that some of the earliest life on Earth flourished in a hot spring environment.
Chemistry in hot springs
But it’s still an open question how complex life formed in these environments. David Deamer, professor of biomolecular engineering at UC Santa Cruz, is trying to answer this question in his quest to understand the origins of life. His search started during a sabbatical in 1975, when he began thinking about the ancestors of membranes, the outer layer of modern-day cells. These early ruminations guided research in his lab for the next four and a half decades.
Conditions on land are ideal for the development of life, according to Deamer. Hot springs — like Bumpass Hell — are perfect: they have the right mix of water and other ingredients for complex chemistry to take place. Also, they often splash water on land, which then evaporates, setting up a series of wet-dry cycles that concentrate molecules found in the freshwater pools. The dehydration process also provides the energy required for condensation reactions — reactions that cause individual molecules to link together to form longer chains.
Bruce Damer, a research associate in biomolecular engineering at UC Santa Cruz, worked with Deamer to directly show that hot spring conditions could drive condensation reactions. In 2018, Damer set up shop at New Zealand’s most active geothermal reserve — ominously named Hell’s Gate. Damer prepared vials with ingredients needed to assemble strands of RNA, a nucleic acid that acts as a messenger during protein synthesis. His concoction included two of the four possible types of RNA nucleotides — building blocks that link together to form longer strands — along with a few other chemical compounds.
Damer placed the vials in an aluminum heat block, roughly the size of two CD cases stacked together. He carefully set the heat block into a near-boiling hydrothermal pool, with towels strategically arranged to keep water from haphazardly splashing into the open vials. To simulate the simulate the sometimes-wet, sometimes-dry burbling of a primordial earth, Damer squirted acidic hot spring water into the vials at regular intervals while carefully kneeling on a wooden board within arm’s reach of the steaming pool. When he brought the vials back to the lab, he found that they contained chains of RNA that were 100-200 nucleotides long. These results, that RNA nucleotides bathed in hydrothermal pools could undergo chemical reactions to connect and form longer chains, support Deamer and Damer’s hypothesis that life on Earth originated in hot springs.
UC Santa Cruz graduate student Zeal Panchal performed similar experiments in the summer of 2019. But rather than crouching next to a bubbling pool of acidic water to warm up a heat block, she used a lab instrument that can be programmed to specific temperatures. Also, rather than using RNA nucleotides, she prepared a solution with the building blocks of DNA. This nucleic acid is more stable than RNA and consequently easier to work with.
To the solution of DNA nucleotides, she also added lipids; oily molecules that come from a class of compounds that don’t dissolve in water, such as fatty acids and waxes. Damer had also added fatty acids to the RNA nucleotide concoction using in his experiments at Hell’s Gate. These lipids form spherical structures called micelles, which are already part of our everyday lives. “When you wash your hands with soap, it foams up, right? That foaminess is due to a structure which the lipid formed, called a micelle,” Panchal describes.
Panchal loaded her DNA nucleotide solution into wells on a glass slide and placed her samples on a heat block set to 80 degrees Celsius. To simulate the cycles of wetting and drying at a hot spring, she periodically squirted water into her samples. And just like Damer observed from his reactions at Hell’s Gate, Panchal’s samples produced longer strands of DNA.
Previous studies have shown that lipids included in these wetting and drying reactions also undergo changes: the oily molecules coalesce to form micelles, shell-like structures that surround the growing molecular chains. Deamer and Damer believe that this process produced protocells, the ancestral precursors of modern-day cells.
Other researchers studying how chemical building blocks hook themselves into longer and longer chains agree that these wet-dry cycles are important. Nicholas Hud, professor of chemistry at Georgia Tech, studies the origins of life from a slightly different perspective: He explores how the ingredients to produce DNA and RNA evolved.
Hud disagrees with Deamer about what the environment would have been like before life began. Whereas Deamer performs experiments using purified RNA and DNA nucleotides, Hud thinks that early Earth was messier and more complicated: He believes that there would have been variations of our modern-day molecules floating around in reactions.
However, Hud agrees that molecules are more likely to link together on land, where wet-dry cycles can occur, rather than in the ocean. The condensation reaction that connects molecules produces water; the formation of such a chemical bond isn’t energetically favorable when there’s already a lot of water around. “The best place to form that is in a hot, dry place. The worst place to form it is in wet, hot place,” he says.
Life at deep sea vents
Other researchers propose that life on Earth originated far from terrestrial hot springs and instead began in the depths of the ocean. Here, heated water spews out of openings on the sea floor, carrying with it a host of minerals collected underground. These conditions can provide the backdrop for exciting chemical possibilities.
“Hydrothermal vents are interesting because they are at the interface of water and rock: you get a gradient there,” says Laurie Barge
“Hydrothermal vents are interesting because they are at the interface of water and rock: you get a gradient there,” says Laurie Barge, an astrobiology researcher at NASA’s Jet Propulsion Laboratory. One gradient is temperature, as near-boiling water hits a colder environment. There are also chemical gradients: some vents expel water that can be as basic as bleach, about 1000 times less acidic than ocean water. These variations — similar to the difference between the positive and negative ends of a battery — provide energy needed to power chemical activity. They can also produce a striking physical appearance: At Lost City, a hydrothermal area in the middle of the Atlantic Ocean, vent fluid reacts with ocean water to produce dramatic limestone chimneys that can rise 30 to 200 feet above the sea floor. These spires are also home to trillions of microbes, feeding off the chemical energy produced by the vents.
To explore the conditions at these underwater locations, Barge creates simulated environments in the lab. “They can mimic what you see in the natural world,” she describes. For instance, in one experiment, she devised an inverted glass bottle that represents an ocean on early Earth: the bottle contains an acidic mixture containing iron, but no oxygen, similar to conditions before life arose. One end of a plastic tube pokes through the lid of the of the upside-down jar, connected to a steady supply of a basic, or alkaline, solution. The result: a do-it-yourself deep-sea vent with a basic fluid spewing into a more acidic, ocean-like environment. Injection of the alkaline solution even produces tiny chimneys, mimicking the formations found at Lost City.
In a 2015 study, Barge and other researchers added RNA nucleotides into an alkaline solution. When they injected the solution into a chimney-producing ocean-simulating environment in the lab, they found that individual RNA nucleotides could link together to form longer chains. These strands were only three or four nucleotides long at most, but the results suggest that the conditions at deep-sea vents could have supported reactions that led to the emergence of life on Earth.
Deamer disagrees that vents were more conducive than hot springs to the earliest stages of life on Earth. First of all, an underwater setting doesn’t support cycles of wetting and evaporation: “There are no wet-dry cycles underwater,” he points out. Furthermore, the concentration of molecules in the ocean would be too dilute for chemical reactions to reliably occur. On land, on the other hand, evaporation would concentrate molecules, such as RNA nucleotides, so there would be enough to react and form longer chains.
Finally, the structure of hydrothermal vents doesn’t allow for gradients to be used in a meaningful way, Deamer claims. For the chemical gradient between vent fluid and ocean water to power reactions, it would need to occur on the scale of nanometers, he says. For example, gradients across cell membranes, which are several nanometers in thickness, can drive condensation reactions that produce energy compounds in cells. Vent minerals, however, create a barrier several millimeters thick. Over those distances, the energy from the gradient would just disappear, Deamer says.
Life beyond Earth
An understanding of how and where life originated on Earth will guide astronomers as they search for signs of life beyond our planet. These biological signatures may be closer than you’d think; there are promising locales only a few planets away.
“One of the things that NASA is really interested in knowing is whether or not there could be life in the subsurface oceans of the icy moons, like Europa and Enceladus,” says Batalha. Scientists have evidence that both Europa and Enceladus — which orbit Jupiter and Saturn, respectively — have oceans of salty, liquid water beneath their icy shells.
These moons are intriguing because they not only have liquid water — thought to be crucial for the formation of life — they also have plumes of water erupting from their surfaces. The Cassini space probe detected signatures from Enceladus’ plumes that there might be ongoing hydrothermal activity. Cassini has even identified compounds containing carbon, nitrogen and oxygen within these plumes; similar compounds can produce amino acids. Europa and Enceladus fascinate astronomers because they resemble the hydrothermal vents found on our own planet and may provide the chemical conditions to support life.
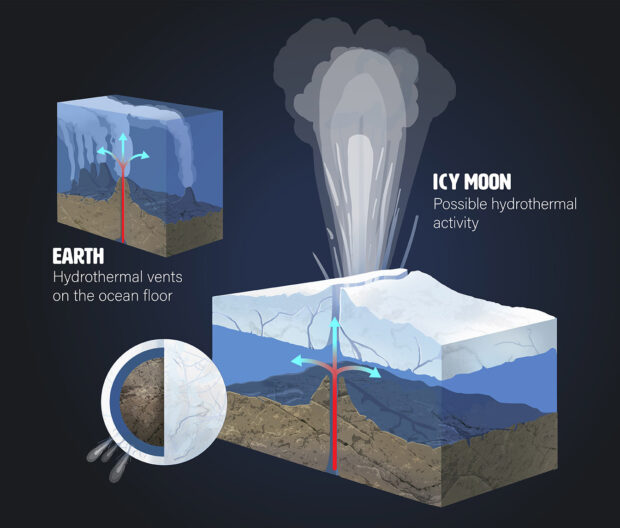
Hydrothermal activity on icy moons could produce conditions like deep-sea vents on Earth. Credit: Madelyn Neufeld / Mady Rose Art
Given his thoughts about the deep-sea vents as the origin of life on Earth, it may not be surprising that Deamer thinks that searching for life on the icy moons may not be fruitful. Instead, he and Damer support a more thorough search on Mars, which has evidence of shallow oceans and volcanic activity. These conditions would sustain the wetting and drying cycles that they believe to be crucial for condensation reactions to occur.
However, Barge thinks that it may still be too soon to write off icy moons.
“Even if you were on an icy moon, you might have freezing and thawing of ice and drying. So, I think it’s important to say, if wet-dry cycling is important, then we should look for any environment in the solar system that might be able to promote oscillating conditions of dehydration,” she says.
Detecting life beyond our planet
One of the reasons that Panchal was working with DNA was to develop a tool for exploring life beyond Earth. She worked with Wenonah Vercoutere, a researcher at NASA’s Ames Research Center, to develop a device that could be used to sequence DNA. Such a device would need to be solid-state — that is, lacking any moving parts — to survive a journey to space. Once there, the device could be used to detect DNA on another moon or planet.
It’s not clear what life would look like beyond our own planet. Mars is millions of miles away from Bumpass Hell, but things may not be so different there, say Deamer and Damer.
“The rules of physics are the same throughout the whole universe. We know that the rules of chemistry, like all the elements in the way that they interact, are the same throughout the universe. So, what is there to say that the rules of biology do not also carry through and are in place and active in the whole universe?” asks Vercoutere.
A version of this story appeared in Science News.
© 2020 Jack J. Lee / UC Santa Cruz Science Communication Program
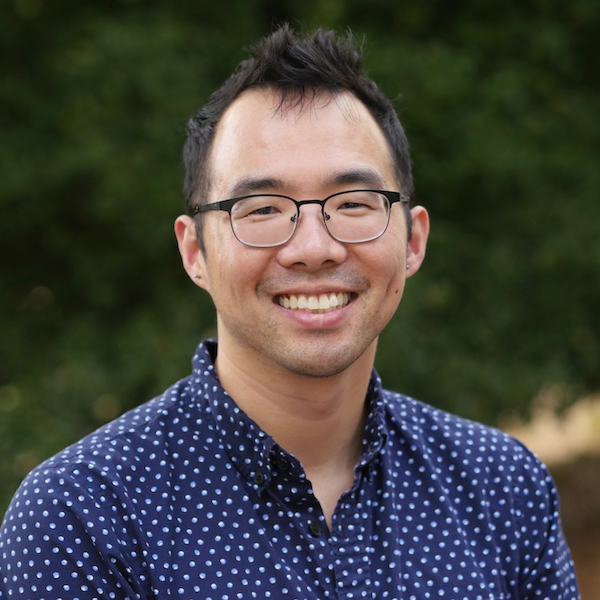
Jack J. Lee
Author
B.S. (biology) California Institute of Technology
Ph.D. (molecular biology) Princeton University
Internships: Science News (Washington, D.C.); American Geophysical Union (Washington, D.C.)
I have a confession: I love making PowerPoint presentations. When I started graduate school, I dreaded assembling figures and writing slides. My attitude changed as I prepared for a talk during my second year and realized that I was simply telling a story. I embraced the challenge of transforming microscopy images into narratives that guided the audience toward my conclusions.
My graduate work revolved around analyzing those images; that experience inspired me to become a data engineer. Though I enjoyed the technical details of the work, I missed the fulfillment of crafting stories. As an aspiring science journalist, I hope to use data to explain scientific news and concepts through a variety of media—maybe even a PowerPoint or two.
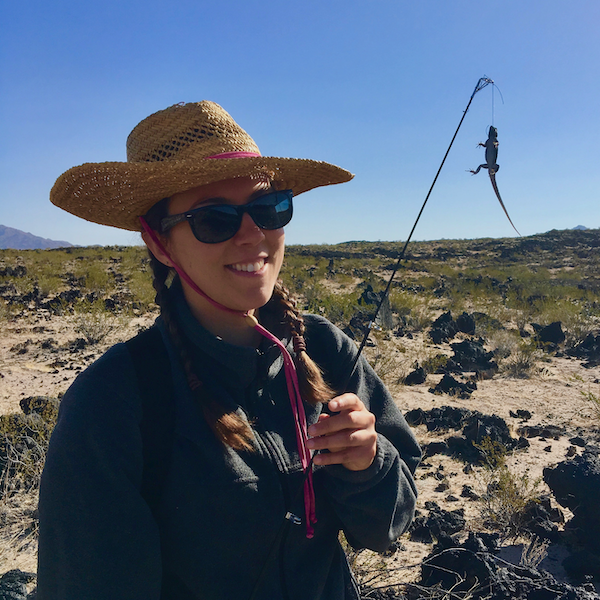
Hannah Caisse
Illustrator
B.S. (ecology and evolutionary biology) University of California, Santa Cruz
Internship: Alf Museum of Paleontology, Claremont, California
Born and raised in Santa Cruz, California, I developed a love for nature at a very early age. Surrounded by the ocean and redwood forests, I became inspired by all of the creatures that were my neighbors. My scientific background is an invaluable asset when I approach my illustrations, and I strive to be as accurate and true to science as possible. My undergraduate curriculum exposed me to a wide array of biological fields, and I was always determined to go above and beyond when it came to incorporating scientific illustration into my coursework. Finishing my graduate studies at CSUMB’s Science Illustration program helped me to formulate my ultimate goal: to generate a greater understanding of the natural world and modern science, one drawing at a time. While not drawing or painting, you can find me reading, baking, rock climbing, gardening, or staring up at the stars.
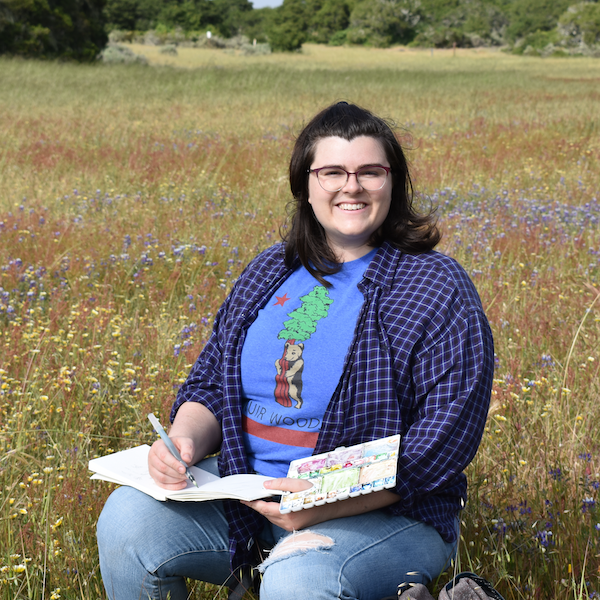
Becca Hoskins
Illustrator
B.S. (ecology and evolutionary biology) University of California, Santa Cruz
Internship: Mahoosuc Land Trust, Bethel, Maine
Becca is currently a Science Illustration intern with the Mahoosuc Land Trust in Bethel, Maine. She loves field sketching and hiking, and is excited to be working on a mural and a local birding guide, as well as informational signage about the natural history of the Mahoosuc Land Trust Properties. She is available for commissioned work, and her portfolio can be viewed at www.BeccaHoskinsArt.com.
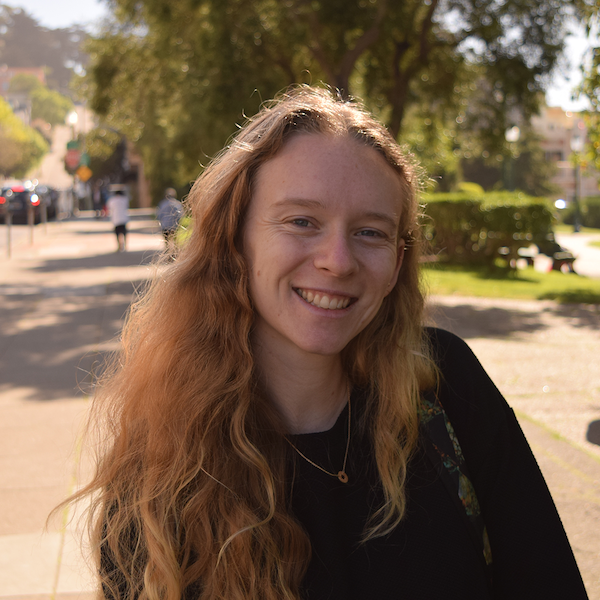
Madelyn Neufield
Illustrator
B.S. (biology) California State University, Fresno
Internships: Morgridge Institute for Research, University of Wisconsin (Madison, Wisconsin); The Smithsonian Institute USDA Entomology Lab (Washington, D.C.)
Madelyn enjoys creating art as a way to share nature’s many curiosities and mysteries. She is currently based in Fresno, CA and collects terrariums full of moss and tropical plants to keep her inspired.
Her passion for nature originally led her to study biology. However, the narrow specificity of scientific research led her to realize that it was nature’s broad diversity that held her heart.
Now, as an illustrator, Madelyn enjoys learning about a range of topics, from the far reaches of space to the tiny worlds of the moss and insects that she collects. She uses visual media to show subjects that are small, far away, unusual, or complicated. Her illustrations highlight diversity in the natural world and explain concepts that cannot be captured in one photograph. Creating artwork inspires her to explore the natural world and she hopes her art inspires others to do the same.