The Twist of Life
An astrophysicist tests her innovative theory to explain why DNA always twists to the right, Zack Savitsky reports. Illustrations by Phillip Callahan and Deborah Besser.
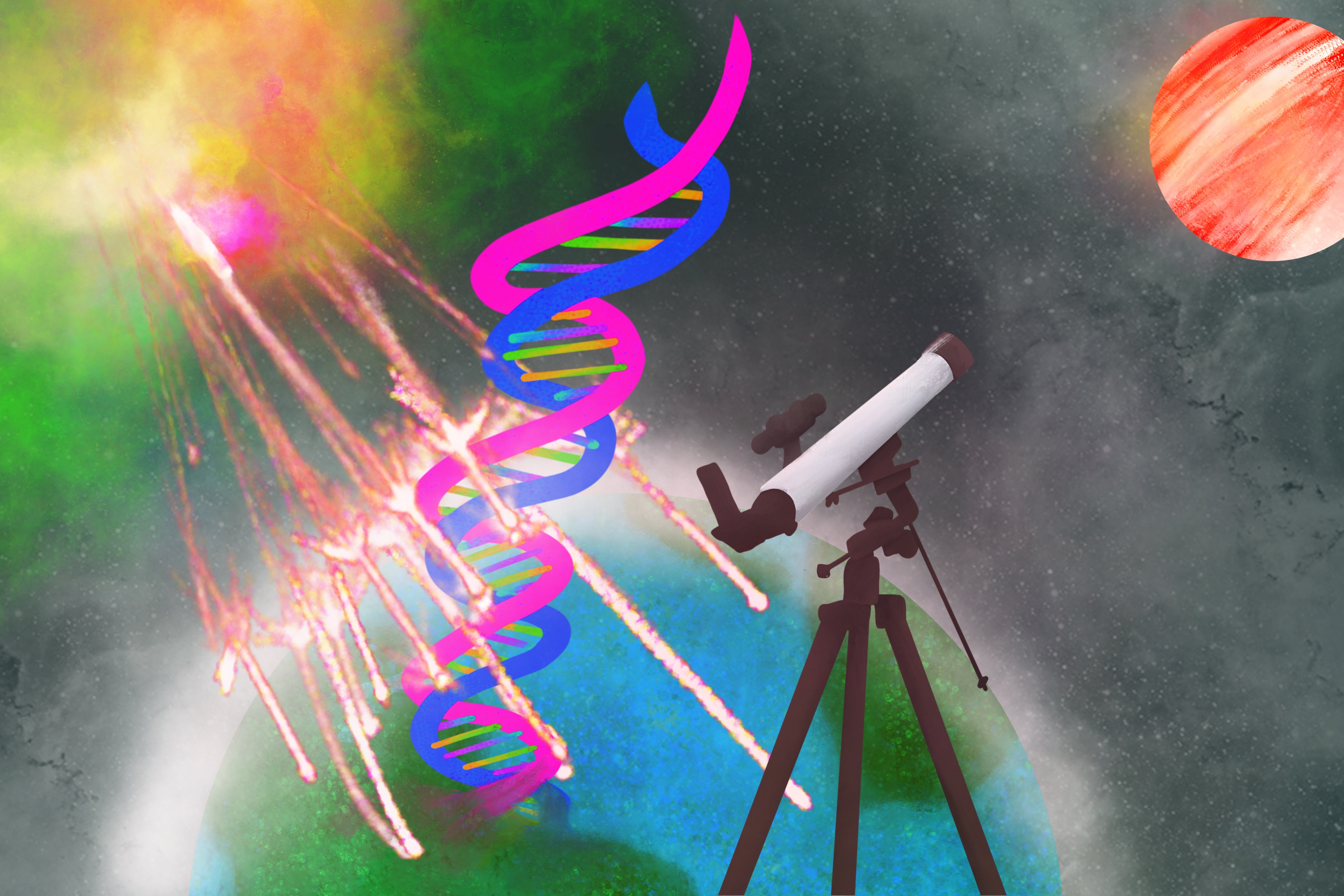
Illustration by Phillip Callahan
A pulsing laser bounces between mirrors and enters a polarizer, which twirls the light like a corkscrew. The photons race down the lab toward a quartz vial of DNA, and a war ensues. Inside the thumb-sized container, strands of genetic code are zapped by light, their base pairs plucked and snapped like strings of a guitar.
Watching intently through protective goggles, a young astrophysicist wonders whether this experiment — the first of its kind — will reveal a cosmic force tuning the instruments of life. If successful, the investigation may help solve a biological mystery that has baffled scientists for over a century: Why does DNA, the iconic double-helix of genetic code, always twist to the right?
Now, Noémie Globus has finally begun testing a theory she hopes will settle the debate. Her series of experiments blasting DNA with different types of radiation seeks to uncover a celestial pressure driving biology’s mysterious twist, and the quest may come to challenge the very definition of “life” — providing crucial insight to the hunt for life beyond Earth.
“The problem with the origin of life is that it seems magic,” Globus said. “It seems that we need so many ingredients, and there are so many different steps that we do not understand. We’re addressing one of those steps — a small puzzle of the big puzzle.”
When jumbled together, molecules of opposing symmetry struggle to perform basic functions of life, such as replicating and metabolizing. So, it makes sense that biological structures have standardized parts — for the same reason carpenters around the world all use screws that tighten in the clockwise direction. With screws, the right-handed design was chosen to make it easier for most people to fasten with their dominant hand. But what about DNA — why does it twist right and not left? Perhaps life developed its signature thread pattern because the universe, as well, has a favorite hand. Globus’s proposal, which builds on decades of discoveries from various disciplines, argues that showers of radiation from the explosions of distant stars and other cosmic calamities struck molecules on the surface of Earth in a way that favored one arrangement of biological nuts and bolts over their mirror version. If it survives multiple rounds of testing, the theory may help explain how life came to be in the first place — both at home, and afar.
Twisty roots
The story begins in a Parisian laboratory in 1848, where 24-year-old Louis Pasteur looked closely at an acid accidentally produced by winemakers that could rotate the direction of light waves — sometimes clockwise and other times counterclockwise. This molecule, he surmised, must be asymmetrical, or “chiral,” with its two mirror versions somehow having distinct properties. Since then, scientists have found chiral molecules galore, and learned to avoid using certain versions — like with the drug Thalidomide, which can either treat morning sickness or cause rare birth defects depending on its form. Both natural and synthetic chemical mixtures typically contain a 50-50 split of the “left-handed” and “right-handed” versions of their chiral molecules — except, curiously, for mixtures produced by living organisms.
In the same city as Pasteur, a century and a half later, Noémie Globus learned about the twisty revelation in an introductory astronomy class. Life, the professor told her, is consistently homochiral (“of one hand”). Amino acids, the building blocks of proteins, are almost always left-handed, while sugars, which form the backbone of DNA, are nearly all right-handed — hence the dependable twirl of our genetic screws. At the time, scientists had no clue how or why living things acquired a regulated design in their machinery.
Globus was hooked. One brief lecture slide planted a seed that would later grow into an obsession, flowering over a decade later as a theory. She toyed around with the question in the back of her mind for years, meanwhile earning a Ph.D. in high-energy astrophysics and studying black holes in Israel. Stationed at an observatory in the French Pyrenees, Globus found herself reading reviews of life’s curious chirality, etching calculations onto loose sheets of paper. Eventually, she came across some studies that connected her biology side-hobby with her astrophysics forte.
For the last hundred years, physicists have often claimed that the universe is governed by symmetries — particles all have anti-particles with opposite charge; a game of pool watched forward and in reverse follows the same laws of motion. Meanwhile, life’s dedication to producing only a specific format of molecules appears as a glaring exception. But there’s one other place where the balance is broken: particle physics. In 1957, Chien-Shiung Wu and her colleagues at Columbia University discovered an anomaly in particles involved with the weak force, one of the four fundamental forces of nature. By cooling down a radioactive form of carbon, she noticed that the decaying particles released electrons that more often flew in one direction than the other, regardless of how the particle’s internal magnet is oriented — a symmetry violation that rocked physics to its core. Not long after, some biochemists began suggesting that perhaps the weak force has something to do with the direction of life’s twist. But the details evaded them.
Star death to stardom
“Life has made a choice to make you what you are — not your mirror image. The question is: Why?”
After wrapping up her work in Israel, Globus moved on to Stanford University, where she worked under the renowned astrophysicist Roger Blandford, a charismatic British theorist with a voice suited for nature documentaries. One day, she mentioned to Blandford the puzzle of life’s construction materials that had long haunted her. She had a hunch that the high-energy space blasts they’d been studying may provide the missing link to explain life’s persistent twist. Once his initial shock and reluctance wore off, the seed took root in Blandford himself, and he encouraged Globus to investigate seriously the origin of life’s homochirality.
“You look at yourself in the mirror, and you see the opposite,” Blandford said. “Life has made a choice to make you what you are — not your mirror image. The question is: Why?”
Over the next few months, the team completed dense theoretical calculations that illustrated, for the first time, a mechanism that connects the anomaly in particle physics to the asymmetry in biology. When giant stars explode, they send out beams of super-energetic particles in the form of cosmic rays, which coast through the cosmos and then collide with Earth’s atmosphere, sparking a chain of reactions that produces secondary particles called muons. That decay process abides by the funky weak force from Wu’s experiment, meaning the muons arrive with their internal magnets pointed only in one direction. These particles, Globus realized, sneak through Earth’s atmosphere and make up most of the radiation on our planet’s surface. Raining down on us constantly, muons occasionally cause our genetic code to mutate. Because they have a consistent magnetic orientation, she thinks, they may generate different mutation rates for right-twisting and left-twisting DNA — helping the former outcompete the latter.
“It was hard to believe that in the rough-and-tumble world of biology this is really going to express itself,” Blandford said. Nevertheless, their calculations showed that the muons were able to generate mutations that favored right-handed DNA — an incredibly slight preference (around one in a million), but perhaps large enough to add up over millions of years. “We were both quite pleased,” he said. “In some sense, it was quite a pretty connection.”
Excited to share their results, the team submitted the paper to journals for publication, but it was rejected. Twice. They even struggled to place the study on a non-peer-reviewed preprint server. The editors, it seemed, were unwilling to believe that an idea so innovative and speculative could possibly check out, Blandford said. “People are vested in the status quo, so they tend to … resist it a bit,” he explained.
Eventually, after a stressful season of applying for research positions with zero publications to show for her work, Globus succeeded in publishing the paper in The Astrophysical Journal Letters. Next came what she called an “emotional lift” — as the team was besieged by press interviews, international media coverage and a slew of collaboration requests from researchers. “It became famous,” she chuckled.
“Oftentimes, especially in particle physics, the effects have no direct consequence for everyday life,” said Martin Bauer, a particle physicist at Durham University in England who helped circulate Globus’s theory among thousands of scientists on Twitter. Globus’s cosmic-ray story offers a relatable demonstration of particle physics at work — an impact so strong that it may aid the search for life beyond Earth. “This would be such a major consequence that’s immediately apparent to people,” Bauer said.
Encouraged by the newfound public support, Globus set out to probe the theory, finding inspiration in unlikely places. At a high-energy physics conference she helped organize in Japan, for example, she watched physicist Junichi Takahashi present his work irradiating biological molecules (in his case, amino acids) with muons — essentially demonstrating a practical way to test her theory. But the talk was entirely in Japanese.
“It was like my dream, right?” she said. “But I couldn’t understand a single thing.”
So, she approached Takahashi after the talk and managed to acquire his business card. Globus, a physical theorist with hardly any lab experience, left Japan newly inspired to put her ideas to the test: shooting pseudo-cosmic rays at DNA to see how life responds to radiation that’s polarized, or arriving with a specific orientation — just like the muons.
“The particle physics part is convincing — there’s no argument there,” Bauer said of Globus’s proposal. “It’s a fascinating theory, and it might well be true, but it’s difficult to prove.”
Step one: securing a laser.
Donning goggles
A few months later, chased out of the U.S. by pandemic-related funding issues and visa troubles, Globus sought refuge with her growing network of international collaborators. She flew to Prague to visit an astrophysics colleague working on high-energy plasma shocks. Inside a sister facility, Globus found a biology lab with a low-powered laser capable of performing her coveted tests.
After describing her theory to the operators, Globus persuaded them to let her conduct a hurried, rather unconventional, trial run of an experiment. She flipped on the laser for half an hour and aimed it at a vial of DNA, which she then tossed in a blue beer cooler and drove across town for another lab to analyze. Unsurprisingly, the imprecise laser measurements and shaken-up DNA sample partially muddled the spectra that she hoped would support her hypothesis.
But while the bootlegged experiment failed to thoroughly assess her theory, it did achieve one thing: “We proved that we could do it,” she said. “We were very happy that we were actually able to make changes in DNA.”
Demo-test out of the way, Globus joined forces with David Deamer, a biologist at UC Santa Cruz who quite literally wrote the textbook on the origin of life. Impressed by Globus’s enthusiasm for her far-out idea, Deamer immediately rattled off various ways they could test it in the lab. Along with the help of physical chemists Eefei Chen and David Kliger and undergraduate assistant Vanessa Mendoza, the duo hopes to finally investigate Globus’s prized theory and offer insight into one of life’s most enduring mysteries.
Ideally, Globus would test her proposal by zapping DNA with a beam of muons. But the highly elusive particles are expensive and hard to come by. So, the team is starting with a more accessible form of radiation: light. Using waveplates that polarize the laser circularly, just as 3D glasses do with light passing through each lens, they can investigate whether radiation not all that different from cosmic rays would selectively affect the mutation rate of DNA depending on which way it’s shaped.
“An experiment is the art of the possible,” Deamer said. “We can do this.”
After the laser does its damage, the scientists will analyze the samples, looking for differences in their structure, sequence and physical characteristics. Using two different types of spectroscopy — circular dichroism and nuclear magnetic resonance — they hope to tell whether the chirality of the molecules is affected by the polarization of the light. Such a discovery would justify repeating the test with more extravagant particles, such as electrons and muons — the ones supported by her calculations.
“Cosmic rays are a bit like red wine,” Globus said. “Small doses are good for you — they give (the population) mutations to adapt. But too many, and you will die.”
But not everyone believes that life was forced into a handedness. Some, like Gabin Laurent, a biochemistry Ph.D. student at ESPCI Paris, assume that there’s no reason DNA chose to twist right over left — there was simply some random imbalance that eventually grew to dominate. His team is searching for a series of complex chemical reactions that could amplify an initial break in symmetry to the near-uniform ratio we see today.
Globus’s proposal has a way around this amplification problem, though. Because cosmic rays routinely bombard the surface of Earth, over generations their small mutating effect on molecules could escalate through the natural evolution of biology. Down the line, she plans to irradiate whole colonies of microbes to test this idea.
“You can’t turn cosmic rays off,” Globus said. “So, we don’t need an extra step; life will amplify all by itself.” If evolution really is responsible for standardizing our screws, maybe this process was a key step in the transition from non-living to living, she added.
The twist is life
This idea — that the universe forced molecules to take up certain shapes in order to mutate and self-replicate — has major implications for identifying signs of life elsewhere.
If it does hold up, the proposal would shine a spotlight on the composer of life’s symphony — helping recognize its tune in faraway places.
On Earth, the only mixtures with one favored handedness are those produced by living organisms. Astrobiologists have argued for decades that homochirality, therefore, could serve as a way to detect life on other planets. Some take the connection even further. “This is a potential definition of life,” said Axel Brandenburg, another physicist-turned-astrobiologist at the Nordic Institute for Theoretical Physics. “It’s such a simple definition, and an extremely remarkable one.”
But there are two main issues with using one-handedness as a biosignature. First, we don’t know what to look for. Life on other planets, in theory, could have DNA that twists to the left. Furthermore, researchers have also measured small chiral imbalances in meteorites — how could we know what’s a disproportion large enough to tell whether something’s actually alive?
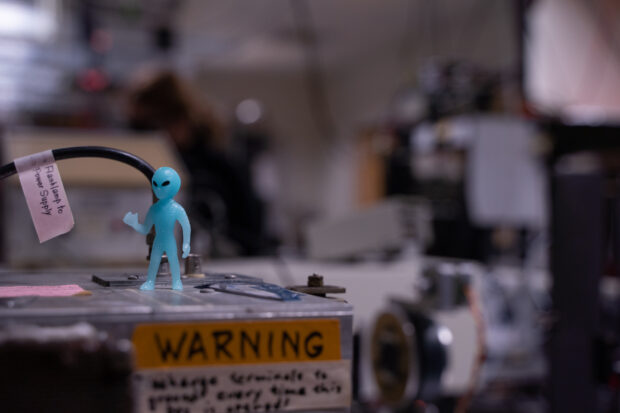
Understanding the origin of homochirality could aid the search for life beyond Earth. (Photo by Zack Savitsky)
The Globus proposal would settle both of these concerns. If her theory is correct, life on other planets should also have right-winding DNA, since it would be subject to the same star-driven polarized radiation as we are. And because her amplification mechanism relies on biological evolution, near-homochirality could be achieved only by living creatures.
“I think knowing the origin (of homochirality) is very important,” said Charles Telesco, an astronomer at the University of Florida. “That would make it much more clear-cut as a probe for life on other worlds.”
Telesco has recently helped design and construct the first chirality-scanning device, known as a spectropolarimeter, that contains no moving parts. After measuring the properties of polarized light, it contracts to the size of a shoebox. “You can take this sucker, fold it up, and put it on a spacecraft,” Telesco said. With it, he hopes, NASA teams will one day be able to look for planets with stockpiles of right-handed sugars, a surefire sign of life, if both Globus and Brandenburg are right.
Globus’s laser test is by no means the end of the line; it will take more than a few experimental results to corroborate her theory. Some, including Bauer, won’t be convinced without further validation outside the lab — like finding consistent chiral signatures around the galaxy.
“You can collect so much evidence that basically there’s no way to come to a different conclusion,” Bauer said. “And I think this is possible if you keep doing these experiments — they’re an important step in this direction.”
If it does hold up, the proposal would shine a spotlight on the composer of life’s symphony — helping recognize its tune in faraway places.
The music of life
While working on their theory, Globus and Blandford began picking up on expressions of chirality all around them. She once sent him a photo of a striped barber pole, joking that cosmic rays must’ve gotten to the blue and red twirls, too. Blandford responded with a link to a catchy song from his childhood: “Misalliance,” by the British duo Flanders and Swann.
Its lyrics tell the story of two star-crossed plants: the honeysuckle, which climbs structures only in a clockwise fashion, and the bindweed, whose vines always wrap counter-clockwise. The two intertwining plants follow the trope of Romeo and Juliet — falling in love, upsetting their parents and realizing that they could never coexist. It concludes:
Together, they found them, the very next day,
They had pulled up their roots and just shriveled away.
Deprived of that freedom for which we must fight:
To veer to the left or to veer to the right!
We’re lucky that life’s molecules, unlike the indecisive plants in the song, twist with consistency. After all, without a standard biological screw, our structures would fall apart, too.
“The interesting question in the song is: Is it a choice that life made? Because this is what we’re trying to see,” Globus said. “Maybe it’s what we’re missing to understand the origin of life — something was driving this.”
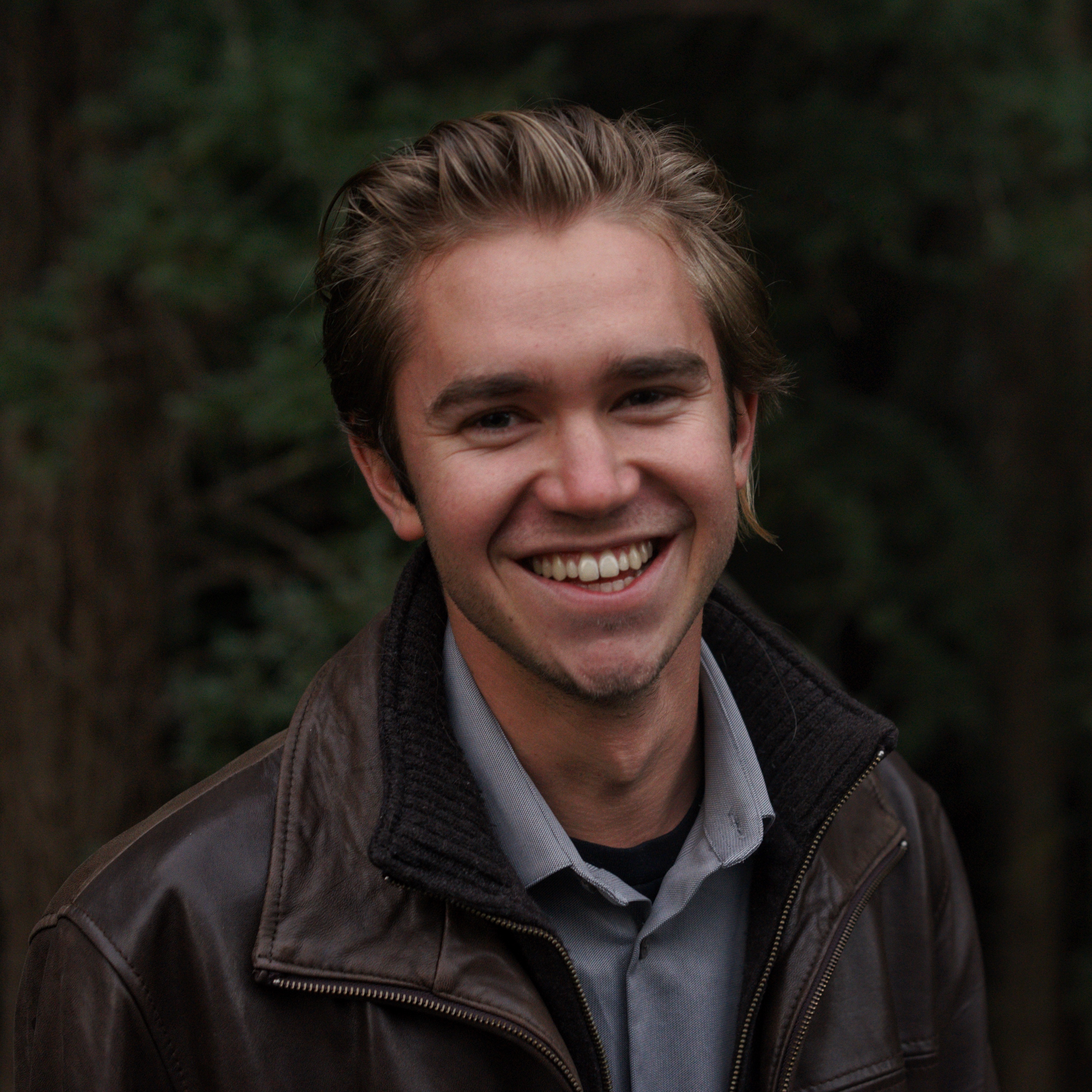
Zack Savitsky
Author
B.S. (public relations; physics, Spanish minors) University of Florida
Internships: Inside Science, The Mercury News, Science Magazine
At age 6, I had my first existential crisis. Just like my garden herbs and hermit crabs, one day, I realized, I would die, and then nothing would matter. Rocked by my premature nihilism, I began to question my whole perception of reality. “What am I made of? Where do those elements come from?” I pondered.
I grew fulfilled piecing together the puzzle of nature—devouring YouTube videos and studying physics. Eventually, I recognized that probing deeper brought meaning to the meaningless. Our unique ability to question, uncover and share fundamental truth is reason enough to live. Enamored by the merit of communicating science, I joined a research lab and founded a club to bring in science writing specialists and link students with opportunities on campus. By connecting puzzle pieces with storytelling, I calm my juvenile terror.
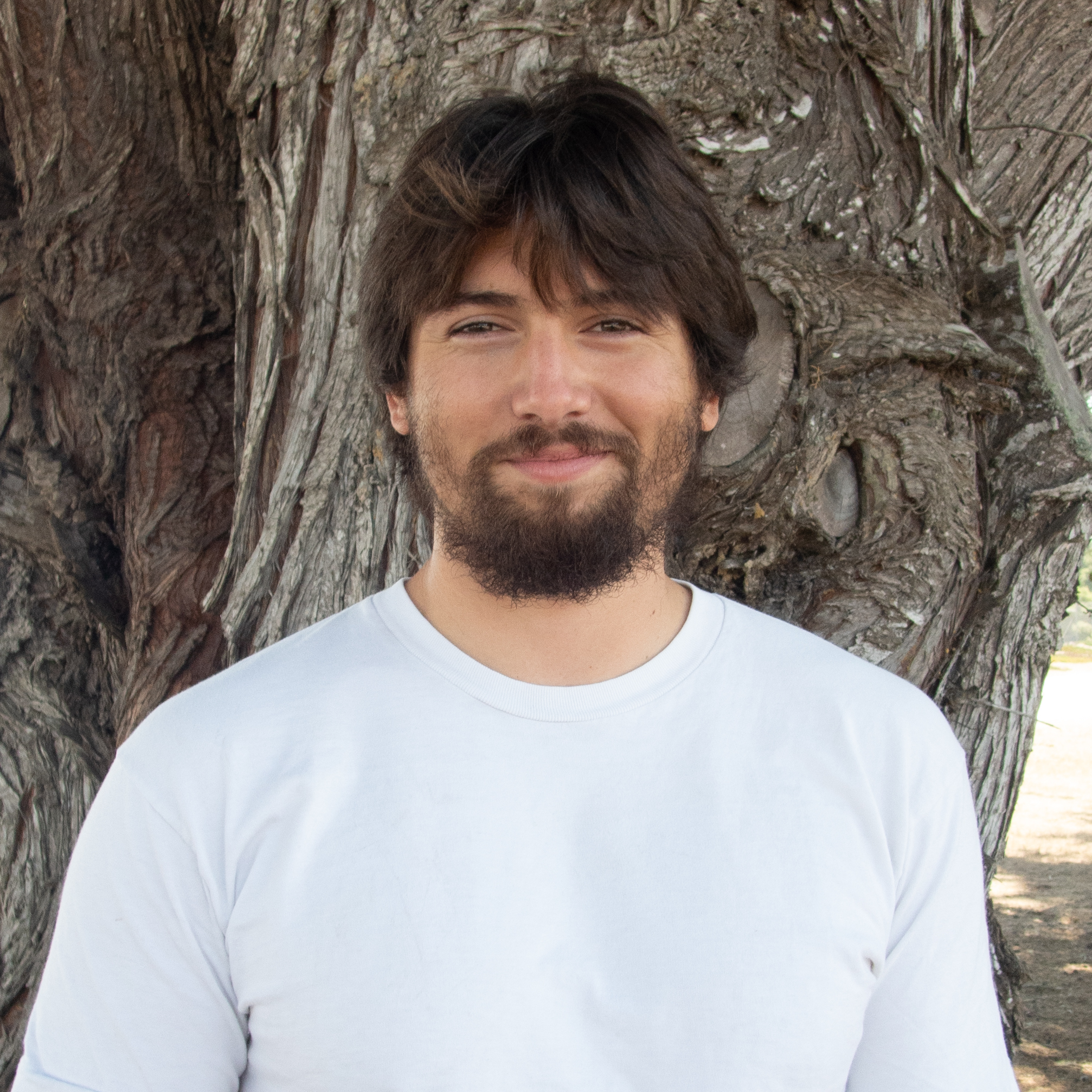
Phillip Callahan
Illustrator
Thank you to all the professors, friends, co-workers, and family members that have continually pushed me past my limits. Without you, I wouldn’t be who I am today, and I will always be grateful for everything. Throughout my life, no matter what avenue I’ve walked down, one thing I always find myself returning to is art. Whether it be expressive, representative or anything in between, I find art to be significant, valuable, and worthwhile. Wherever this life takes me I will always continue to engage in creative work.
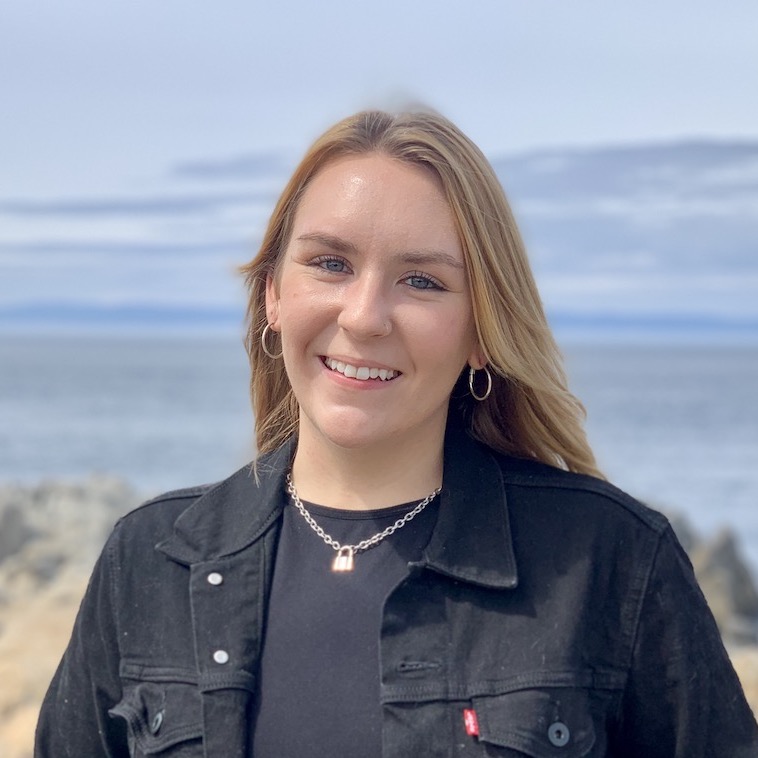
Deborah Besser
Illustrator
B.S. (biology) Santa Clara University
Internships: Hawaii Institute of Marine Biology, Upwell
“Yep, you were made to be a scientist.” In childhood, this was my mother’s patient response whenever I barraged her with questions about the natural world. The prospect of being able to go into nature and answer those questions myself led me to pursue a biology degree with hopes to enter the research field. But art has always been equally important to my identity because it helped make the natural world more digestible- if I couldn’t understand something, at least I could try drawing it and learn something new in the process. For a while, I felt torn over the thought of compromising one passion for another, until one class introduced me to the world of science illustration. In this class, bench labs were replaced by the creation of a field journal, and that journal set me on a new path. I finally got to integrate my interests and was captivated. I still take that journal with me on hikes and camping trips because field sketching remains one of my favorite ways to connect with nature, as it always has been. It reminds me of where I started and gives me hope for the new paths that lie ahead.