Thinking outside the body
A materials scientist is making connections in cell biology, and Laura G. Shields heads deep inside to find out how. Illustrated by Chen Zha and Yun-Kae Kiang.
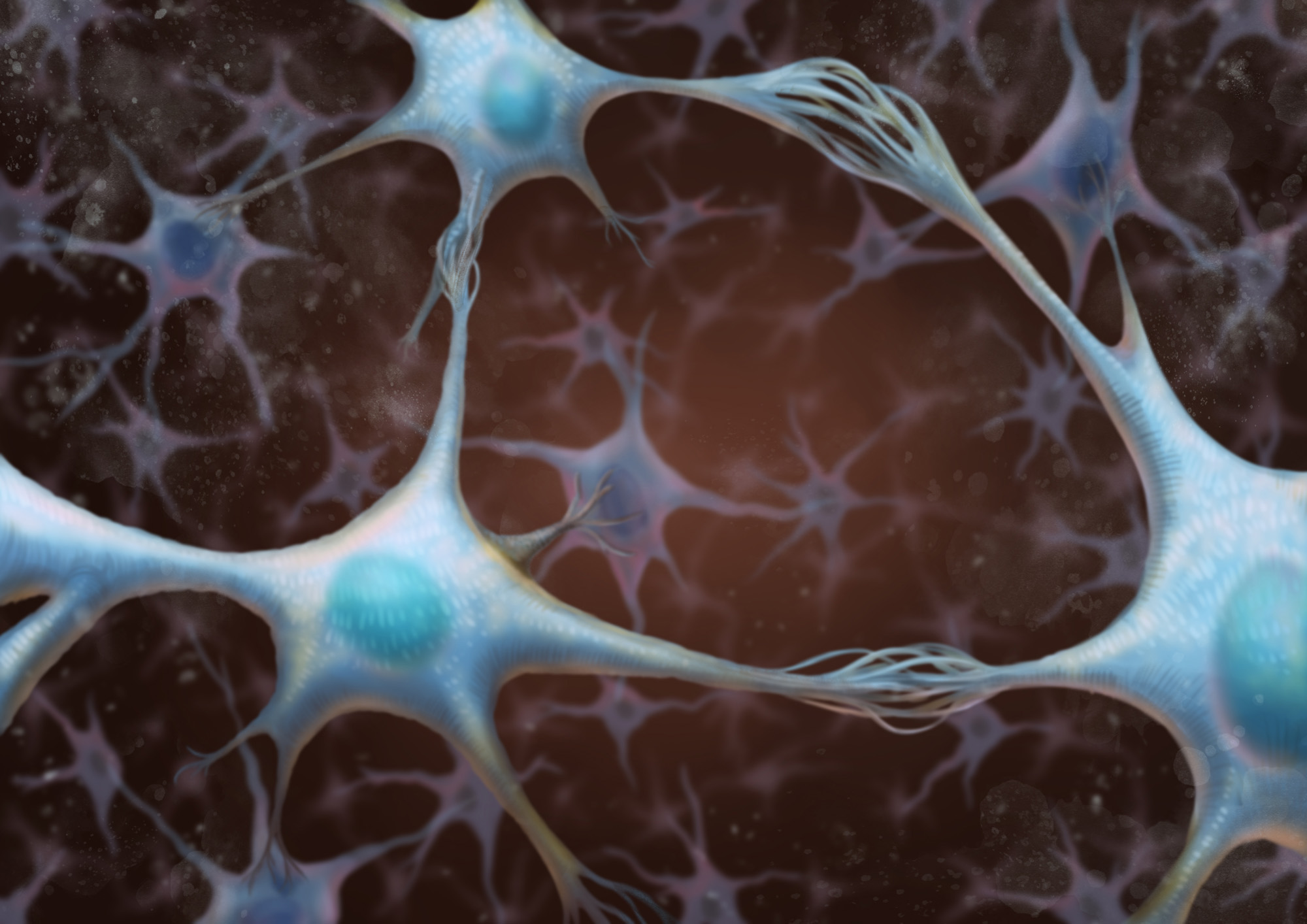
Illustration: Chen Zha
For decades, cell biologists struggled to keep human cells alive outside a person. But then came Henrietta Lacks. Lacks’ aggressive cervical cancer cells were the first that scientists were able to grow in the lab. These cells – named HeLa – starred in groundbreaking landmarks in modern medicine.
Researchers can now culture many different types of cells, including human cells, says Sarah Heilshorn, a materials scientist and engineer at Stanford University. Yet as in the pre-HeLa days, today’s common cell culture technology blocks scientists from asking some sophisticated questions about the lives of cells.
“We culture them on a petri dish. It’s just a flat dish,” she explains, sketching a flat surface with her hands. “They’re isolated little cells, and they’re not doing anything like what they do in our bodies.”
Biologists now want to coax cells into systems that better resemble human tissue. So, in the past decade, they have started growing cells in three-dimensional (3D) structures. Heilshorn is helping them build these structures.
If the cells are like the bricks of a building, they must be stuck together by mortar – a material called the substrate. Heilshorn’s research group is designing better substrates and testing how they affect cell behavior. Her gel-like materials are adjustable; by tuning their properties, Heilshorn can learn more about cell biology. She’s already used this approach to discover how to help some cells, such as brain cells, grow better in 3D. One of her goals is to allow researchers to create better mimics of human tissue outside the body – in a setting known as “in vitro.”
Such tissue mimics could transform biology and medicine. “A robust cell-based in vitro model that recapitulates key aspects of human disease has the potential to accelerate our understanding of human disease,” says Finn Hawkins, a pulmonologist with a lab at the Center for Regenerative Medicine at the Boston University School of Medicine.
Stemness
Medical researchers are especially interested in growing stem cells, whose stemness – or ability to both self-renew and to form all different kinds of cells – has made them invaluable to understanding how tissues develop. But stem cells are tricky to grow. Researchers want to culture stem cells in their undifferentiated, or unchanged state. If the cells begin to differentiate into more specialized cells, such as brain or lung cells, they lose their stemness, and scientists have difficulty coaxing them into the desired cell types.
Scientists at the University of Wisconsin-Madison extracted the first human embryonic stem cells that were kept alive in the laboratory two decades ago. Since then, scientists have had an easier time maintaining stemness in 2D cultures, where they can move freely, than in a 3D environment, where the cells have less freedom to roam.
“Stem cells especially should receive the same kind of environment that they see inside the body or the environment that they’re going to differentiate into,” explains Prashanth Asuri, a bioengineering professor at Santa Clara University.
In 2009, Hans Clevers at the Hubrecht Institute in Utrecht, the Netherlands, developed the first human organoid – a collection of cultured cells that mimicked the intestines. Organoids are tiny, simplified 3D versions of an organ produced outside of the body. The achievement kicked off a race to develop organoids modeling the other major organs, including the liver, breast, eye, and brain. Most laboratories used the same substrate in which Clevers found success. It’s called Matrigel, and it’s a gel derived from mouse tumors.
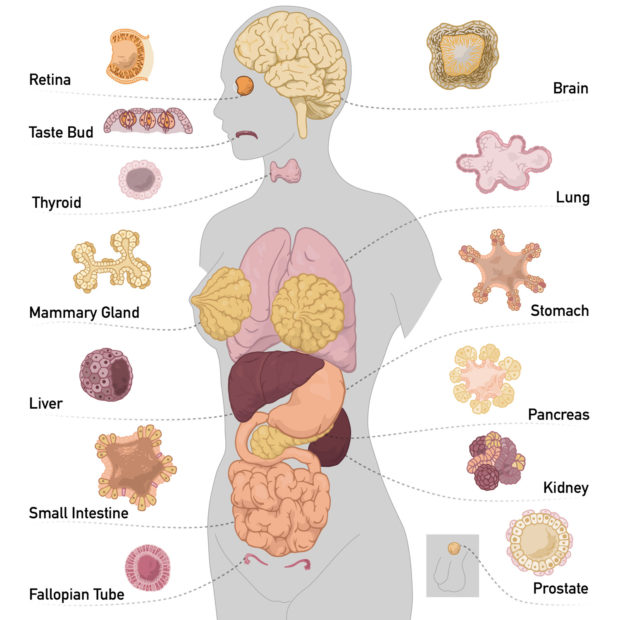
Researchers have developed organoids that model many major organs of the human body. Illustration: Yun-Kae Kiang
But Matrigel is not perfect. This stew contains 2,000 or so different compounds, and there’s a lot of variability among batches, which creates problems, explains Ruby Dewi, a biologist in Heilshorn’s research group.
So researchers, including Heilshorn, have started developing artificial gels, called hydrogels. Importantly, they know exactly what they’re putting into these hydrogels. “We try to make materials that in some way mimic those that are found in our own bodies,” she explains. Her group starts with proteins, one of the most common building blocks in the body.
Multifaceted approach
Heilshorn is well suited to tackle this interdisciplinary problem. Encouraged by her high school interests in chemistry and mathematics, she pursued chemical engineering as an undergraduate student at Georgia Tech. After entering graduate school at Caltech in 1998, a talk about nature-inspired biomaterials caught her attention. The speaker, chemist David Tirrell, ultimately became her PhD adviser.
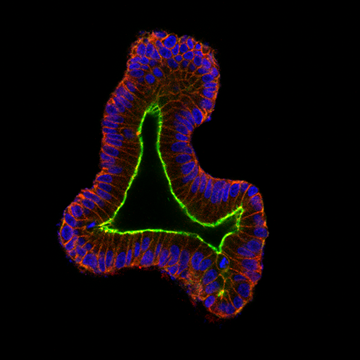
An organoid, developed in Heilshorn’s laboratory, modeling the human small intestine. Photo: Dan Hunt
Around that time, a new generation of biomaterials emerged. Scientists had used inorganic materials, such as metals, as implants in the human body. But now researchers could design biomaterials encoded with biological information that could guide the behavior of the surrounding cells and tissues.
Heilshorn thought she didn’t know enough biology to work with these biomaterials, so in graduate school, she taught herself biology, catching up with undergraduate biology classes.
Her graduate research ultimately covered chemistry, physics, bioengineering, and culture and cell biology. She expanded her reach into yet another area – neurobiology – for her post-doctoral research at UC Berkeley, investing her time and energy into stem cell biology. She chose that field specifically, she says, because being in California gave her funding and access to stem cells – resources that were more uncertain in other regions at that time.
“It was clear that Sarah had the breadth to be able to work across that range of disciplines,” says Tirrell. “And I think she’s done that even more successfully and more ambitiously in her own research group at Stanford.”
Still, the basis of her training and identity is engineering. Engineers, who are “reductionists by nature,” have a different approach than cell biologists, according to Heilshorn. “We want to make as simplified a model as possible to capture some biological phenomenon that we think is important,” she says.
Tuning the matrix
To better understand how the properties of the 3D material influence cell stemness, Heilshorn and colleagues designed a family of 3D hydrogels from proteins with tunable mechanical properties. This range allowed the researchers to make small changes to the gel’s properties — endowing them with more flexibility, for example — and then systematically test how it affected a specific stem cell population.
Past studies had earmarked matrix stiffness – basically, the rigidity of the gel – as an important factor in maintaining cell stemness. But the researchers surprisingly found stiffness had little effect on stemness for the neural stem cells they examined. Instead, the researchers found that cells tended to retain their stemness better in the gels that more easily break down and remodel, a property called degradability. The team published their results in December 2017 in Nature Materials.
Unlike other studies on biomaterials, Heilshorn’s group “had this capacity to intelligently design the biomaterials,” says Asuri. Generally when working with biomaterials, changing one variable causes another property to change as well, so it’s difficult to test one property without changing others. But Heilshorn’s approach allowed the team to independently study stiffness and matrix remodeling, Asuri says: “That’s just fantastic.”
As it turns out, physical contact between the neural stem cells helps them retain stemness, explains Heilshorn. The researchers found that only once the neural stem cells could touch each other would they divide and make copies of themselves. If the properties of the gel prevented cell connections, the cells couldn’t grow and divide as they normally do in the body.
The engineering of the material allowed us to identify something about the biology.
“The engineering of the material allowed us to identify something about the biology,” says Heilshorn.
The results are encouraging other researchers to try growing organoids in new materials. Madeline Lancaster, a developmental biologist at the MRC Laboratory of Molecular Biology in the United Kingdom, studies the basic biology of human brain development. Lancaster says that Heilshorn’s study “looks very promising because of [the material’s] ability to allow neural stem cells to behave as they would in an actual brain. That’s definitely something we should try.”
A better way to test
Heilshorn is excited to take her biomaterials to the clinic, so they can help patients. She recently started working with Stefanie Jeffrey, a cancer researcher at the Stanford University School of Medicine, to test whether her materials could help the search for new cancer treatments. Cancer cells that survive initial drug therapies evolve and become resistant to standard therapies, Jeffrey explains. She’s eager to use Heilshorn’s gels to develop human-based mimics, so she can test new drug treatments that might attack advanced cancer cells.
Drug developers hope that better mimics will help them make better drugs, faster. It costs $2.6 billion to get a drug approved, partly because failed trials are so expensive. One major reason why drug trials fail is that they’re usually tested in animals before humans, and animal models don’t do a good job of predicting how well a drug will work in humans. Heilshorn’s research could help address this problem.
“If you had cell-based assays to identify which ones are the most promising, human cells might be a way for accelerating drug discovery,” Hawkins says.
“The biggest strength of these in vitro models is that they are human models,” Lancaster adds.
Doctors could ultimately look at such models for individual patients to inform their personalized treatment decisions. That drives Heilshorn. “The ability to impact patient outcomes rapidly,” she says; “there’s just so much more potential in this direction.”
© 2018 Laura G. Shields / UC Santa Cruz Science Communication Program
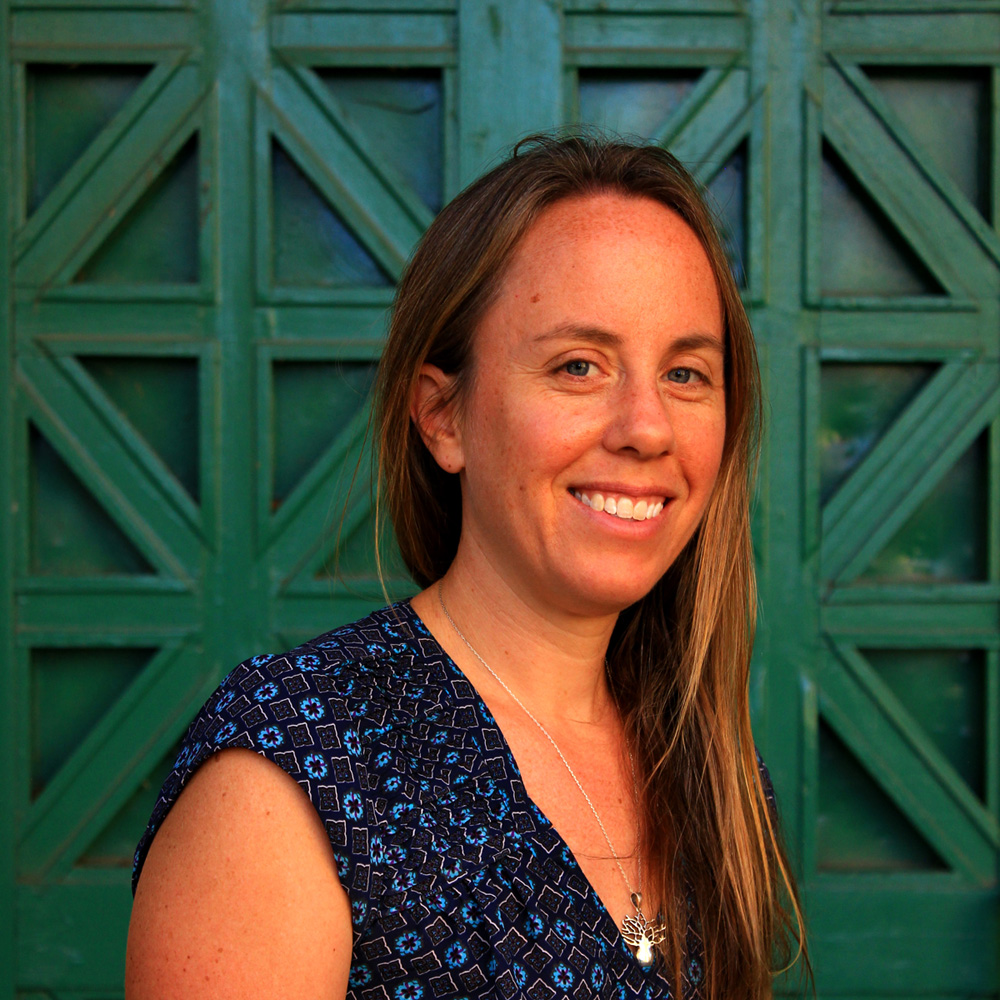
Laura G. Shields
Author
B.S. (chemistry) University of Virginia
M.S. (chemistry) University of California, San Diego
Ph.D. (chemistry) University of California, San Diego
Internship: Pacific Northwest National Laboratory, Richland, Washington
As an eight-year-old, I wrote a newsletter warning my neighbors that pollution was ripping a hole in the ozone layer. I never distributed the newsletter, but still wanted to educate others about environmental problems; I just needed to find a better way.
In high school, I fell hard for the world of atoms when I realized that unlocking the secret code of chemistry explained everything around me. I later studied how pollutants such as nitrogen dioxide, sulfur dioxide, and particulate matter harm air quality, merging my passions for chemistry and environmental issues.
In Australia years later, I patrolled beaches to count nesting sea turtles. When I wrote a travel magazine article about how poaching and other threats hurt these creatures, I realized I had finally found a better way to share science.
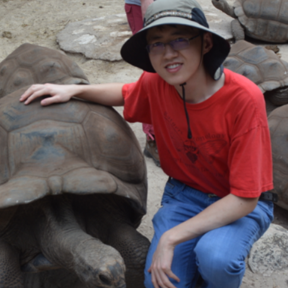
Chen Zha
Illustrator
B.S. (biology) University of Science and Technology of China
M.S. (entomology) North Carolina State University
Ph.D. (entomology) Rutgers University
Internship: Smithsonian National Museum of Natural History, Washington, D.C.
Chen is a science illustrator with a scientific background; he graduated with a Ph.D. in entomology at Rutgers University before he started an artist’s life. Being an insect enthusiast from childhood and working in entomology for 6 years, Chen is also a generalist with broad interests in all living things and scientific subjects. His goal is to promote science education and communication with visualized information and show the beauty of animal groups that have received little attention.
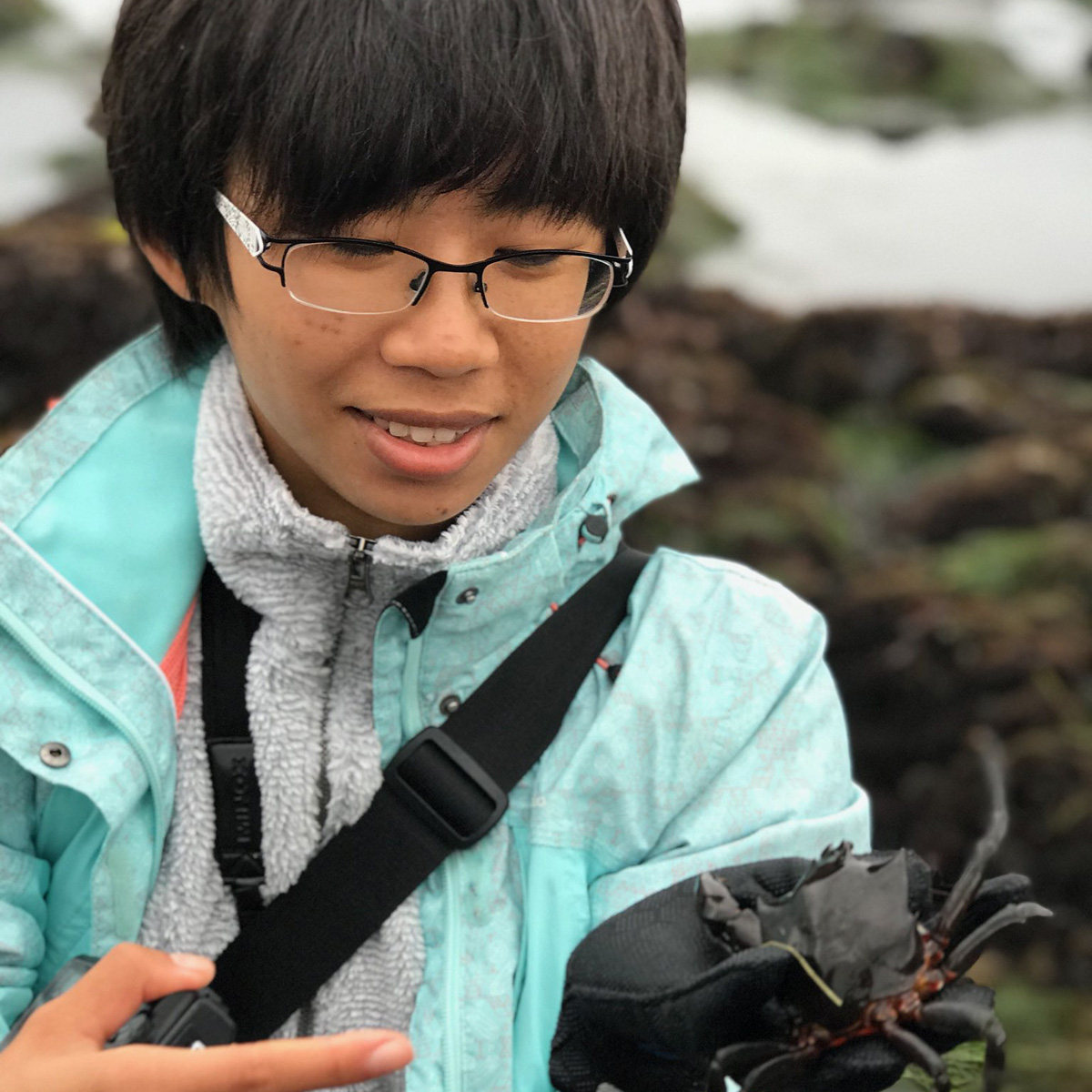
Yun-Kae Kiang
Illustrator
B.S. (life science) National Taiwan University, Taipei, Taiwan
M.S. (marine biology and fisheries) National Taiwan University, Taipei, Taiwan
Internship: Smithsonian Tropical Research Institute, Panama
Yun-Kae is a naturalist and artist from Taiwan. Her passion for science originated from an obsession with marine and tropical ecology, and it has extended into a wide spectrum from oceanography to indigenous cultures. She enjoys doing experiments and explorations to confirm hypotheses and discover phenomena. With a curious and creative mind, she is eager to unveil the little-known natural world to all of you—unconventionally, from science illustrations, designs, and sculptures, to crafts.